Nov 02, 2010For the past two years, researchers at the University of Southampton have observed conditions within a glacier in the mountainous reaches of Iceland from their office in the United Kingdom, thanks to a prototype wireless sensor system that the group is testing. During that period of time, however, the system has suffered from data interruptions and shutdowns, caused by power failures or harsh winter weather. Next year, the research team aims to further improve the system with additional battery power for the sensor nodes, as well as a Wi-Fi system for more reliable, faster connectivity to software running on a back-end server.
The wireless sensors system (dubbed Glacsweb) is intended to help the scientists track the behavior of glaciers as they melt and move over time, by measuring the ice sheets' motion, angle and temperature, and then transmitting that information to software that can be accessed via the Internet. The ultimate goal is to learn more about glacial "stick-slip" motion, in which ice flows in phases—sticking for a time, and then moving quickly through a slip phase. The technology, custom-built by university researchers and installed two years ago on Iceland's Skalafellsjökull Glacier, included eight sensors, only three of which are currently still transmitting.
In the coming year, the researchers plan to use new more sensitive sensors to detect ice quakes (concussions as a result of ice breakup), and will attempt to transmit that data from the base station via Wi-Fi, using a Wi-Fi antenna mounted at a neighboring Icelandic farm. In addition, the project's principal investigators—Kirk Martinez, a professor in the university's Department of Electronics and Computer Science, and Jane Hart, a geography professor at the college—deployed a similar system this month near Tijuana, Mexico, to monitor ground movement in an effort to predict future mudslides following heavy rains.
Martinez and his research team first began studying wireless sensor systems for monitoring glaciers in 2003, in Briksdalsbreen, Norway. He and Hart were seeking a system for remotely sending data about glacier dynamics throughout the year, without using cables, which typically snap under the pressure of heavy moving ice. Wireless sensors can move with the ice, and are thus more robust. The group drilled 260-foot-deep holes in the ice (the melting ice resulted in a subsequent annual loss of more than 30 feet of depth), and dropped the sensor probes inside. On the glacier's surface, they erected a base station, consisting of a computer wired to a transceiver, in order to receive transmission from the wireless sensor probes. The computer uses software designed by the researchers to interpret that information and transmit it via GPRS (the nearest cell tower is six miles away) to the Glacsweb software (also designed by the research team), operating on a server hosted by the University of Southampton.
While the scientists initially employed 868 MHz battery-powered wireless sensor probes, they were unable to transmit on warmer days through pockets of air and water. Therefore, they switched to using 433 MHz active transponders (utilizing proprietary transmission specifications), "which worked quite well," Martinez says. The sensor probes were designed to transmit a 100-milliwatt signal—powerful enough to pass through the ice and water and reach the base station. The system was still operating four years later, and had produced sufficient data for researchers to begin evaluating how much the glacier had moved and been reduced, and thereby proving the technology worked.
In summer 2008, the team took additional sensors and a base station to a colder, more challenging environment, at Skalafellsjökull. At that site, the glacier measures 328 feet deep and 30 miles in length. This time, the sensor probes transmitted at 173 MHz—by using the lower frequency, researchers were able to reduce the loss of transmission through scattering and reflection—again using a proprietary standard. Like the probes utilized in Norway, these included temperature sensors, accelerometers, electrical conductivity (to detect liquid water, as opposed to ice) and strain sensors. A GPS receiver mounted on the base station itself (which stood on the ice, above the probes) provides data indicating the direction and speed of the glacier movement. The probes and GPS receiver take hourly readings of latitude, longitude and sensor data, including temperature, movement (such as tilting and turning) and pressure, then transmit that data once daily, at around noon.
As in Norway, the base station transmits data once daily, via a GPRS cell tower located not far from the project site, to the server in the United Kingdom, where the software (designed to receive, interpret, store and display data from the sensor probes on a server accessible to researchers) calculates any change in sensor readings from the most recent transmission—including the location of the glacier base station, based on the GPS coordinates. A second base station, located a half-mile away on dry ground, takes a static differential GPS (dGPS) reading, which is also forwarded to the server via GPRS, to be synchronized with the information from the base station regarding the glacier above the probes, thereby calculating the glacier base-station's location. By pinpointing the station's exact location via GPS technology, the movement of the ice on which it stands can also be monitored. In addition, the sensors can receive instructions from the back-end system, such as a request to report sensor data again, or to carry out tasks such as measuring the received signal strength from the base station transceiver, in order to determine the system's health.
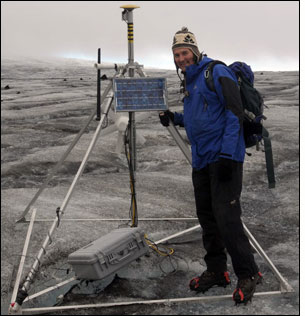
The glacier base station is powered by a wind turbine and a solar panel, which together can provide as much as 30 watts of power.
The Web-based software in the back-end system enables information to be made available to online users. Glaciologists have been publishing information based on the sensor data regarding how the ice's movement has been affected by rain.
The GPRS system has had some shortcomings, Martinez says, noting that the system's reliability was affected by weather when transmitting, and that there were very frequent signal dropouts and a low data rate. Researchers visiting the glacier site had to crouch down in the ice for several minutes to avoid interfering with the daily GPRS transmission, and an additional GPRS antenna needed to be installed to ensure that data was properly sent.
In a new phase set to commence in spring 2011, the group intends to begin testing new sensors that will transmit more frequently, thanks to greater battery power. These new sensors will create a mesh network with probes passing along signals from one to the other, until the data reaches the base station on the ice's surface. The reader, in turn, will transmit the data to an Internet-connected Wi-Fi router at a neighboring farm.
Earlier this month, Martinez and his team installed a similar system with three sensors in Tijuana, in order to allow California scientists to determine if they could detect landslides based on the behavior of mud following a heavy rainfall. The Tijuana project is being funded by the National Oceanic and Atmospheric Administration (NOAA), in the United States. In this case, the sensor probes, buried 50 centimeters (20 inches) in mud, are transmitting at 173 MHz to a base station (with a transceiver and a computer mounted on a tripod) that then forwards the data via GPRS—but in this scenario, the sensors are transmitting every hour. HoundSystems is providing the software to manage the sensor nodes and interpret their data, as well as the server on which the software also analyzes the results and then makes it available to the public on the San Diego Coastal Storms Web site.
The University of Vermont's department of computer science and school of engineering has developed a somewhat similar wireless sensor system. The technology has been used at Sagehen Creek Field Station, a research facility in Truckee, Calif., operated by the University of California, Berkeley. Six 2.4 GHz active mesh-network transceivers were embedded in snow to measure snow depth and snow water equivalence. The wireless system has been used in conjunction with manual measurements, says Christian Skalka, a University of Vermont associate professor who is heading up the project, and is now being compared against those measurements—thus far proving to be just as accurate, he indicates. Data from the sensors is being received by a base station and then forwarded to a server at the University of Vermont, where the researchers' software interprets and stores information about the snow.